Optics and Photonics Applications Laboratory (OPAL)
Photonic technologies are proving transformative for a wide variety of industries and academic fields.
Micron-scale ring resonators, in particular, have been crucial for advancements in biosensing and immunology [1], optical communications [2], quantum computing and informatics [3], and as components of integrated circuits with a variety of applications [4]. In line with the vision of the National Academies’ Decadal Survey for astronomy [5], our research seeks to solve the engineering problems associated with the application of photonic technologies to astronomical instrumentation. Because observations of stars, galaxies, or other astronomically interesting phenomena in the wavelength range 1.0 < λ < 2.5μm are hampered by the forest of emission lines in the upper atmosphere generated by OH (hydroxyl) molecules (Figure 1), technologies for eliminating such background interference are crucial to the success of ground-based infrared astronomy. For example, observation of narrow-band emission from positronium [6] require the suppression of just a few adjacent OH lines, while supernovae at cosmological distances can benefit from the suppression of tens (or even hundreds) of lines overlapping with their broader spectrum.
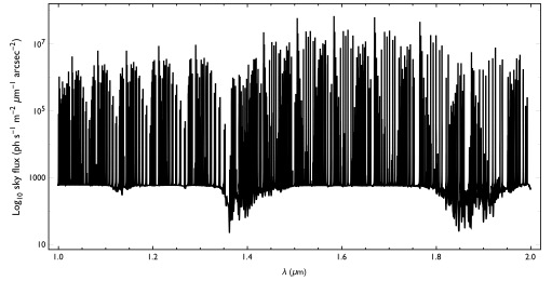
Figure 1: (Left) A model spectrum of the near-infrared night sky dominated by OH emission lines. (Right) The OH lines are intrinsically very narrow, with dark sky inbetween.
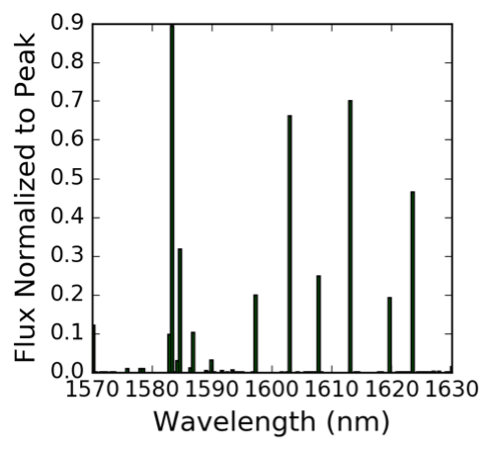
We, therefore, have chosen to focus on the development of ring resonators as the foundational technology to realize OH suppression in infrared ground-based astronomical instrumentation. Theoretical estimates show that suppression (by 20-40dB) of a handful of the brightest OH lines by ring-resonator-based notch filters would improve observed signal-to-noise by a factor of five or more [7]. This means that – barring other sources of noise or signal loss – telescopes of even modest (1m) aperture size can improve their performance to match that of a much larger aperture (5m) telescope!
The goal of our research is to resolve heretofore-intractable engineering problems with ring resonator-based notch filters, improving their performance to the point where they can be usefully integrated with functional astronomical instrumentation.
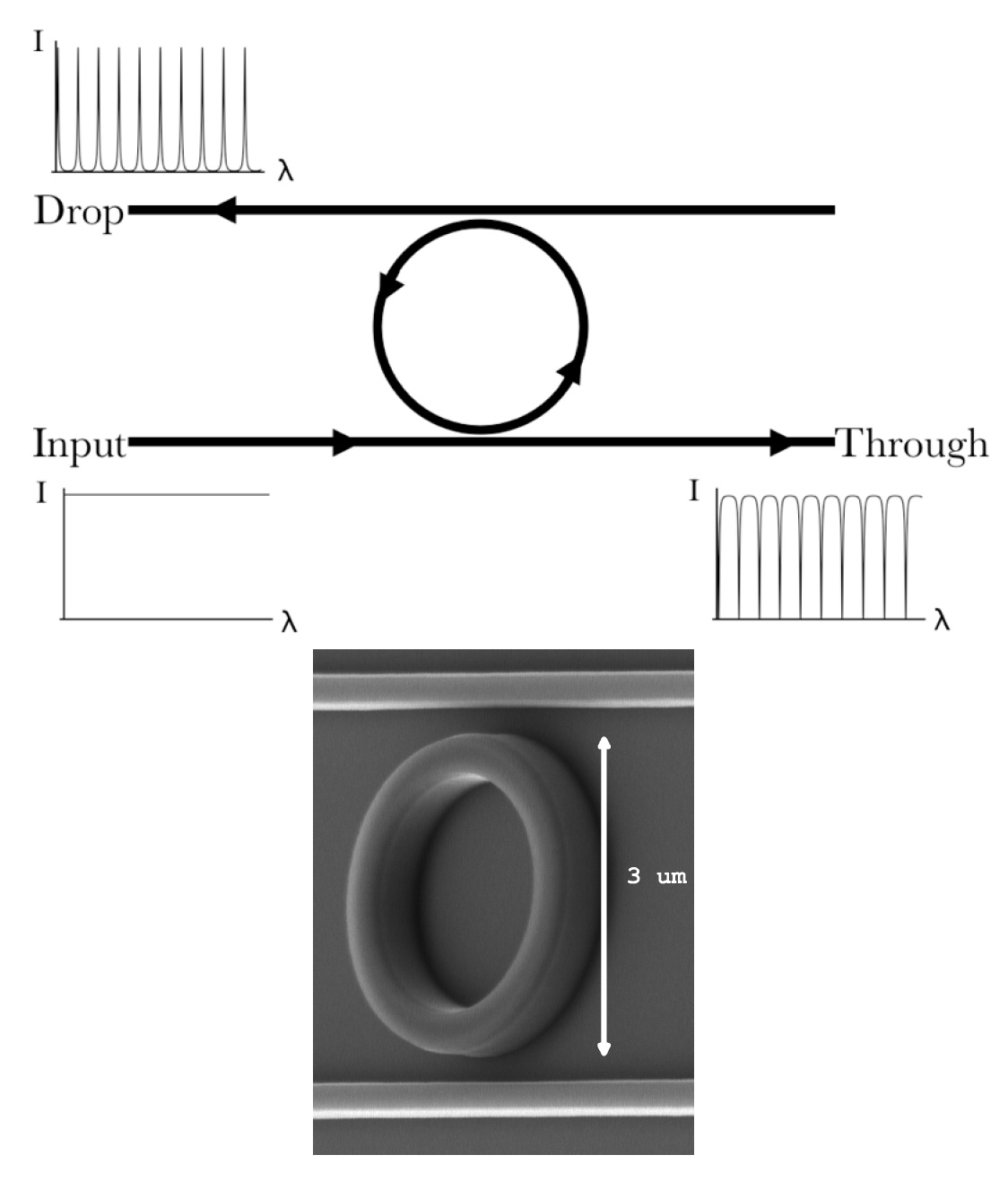
Figure 2: (Top) A schematic diagram of a simple ring resonator showing the input, through and drop ports and a sketch of the spectrum at each port. (Bottom) SEM image of a prototype silicon photonic ring resonator with through and drop port, manufactured at the Center for Nanoscale Materials at Argonne National Laboratory.
Ring resonators are lithographically printed on silicon wafers and coupled to waveguides for signal propagation. As light travels down a waveguide adjacent to a ring resonator, specific wavelengths – those that match the circumference of the ring: nλ = πD couple to the resonator and are “dropped” from the signal that is propagated to the through port and the output fiber – see Figure 2. Meanwhile, the depth of the notch filter (measured in dB) depends on the quality of the lithographic fabrication process of the ring, as well as the efficiency of the coupling between the waveguide and the ring (which is in turn dependent in part on the physical separation between the two components).
Results of Prior Research
Our collaboration has been pursuing ring resonator-based notch filters as an engineering solution for OH suppression for eight years. Our investigations to date have coalesced around a five-step process:
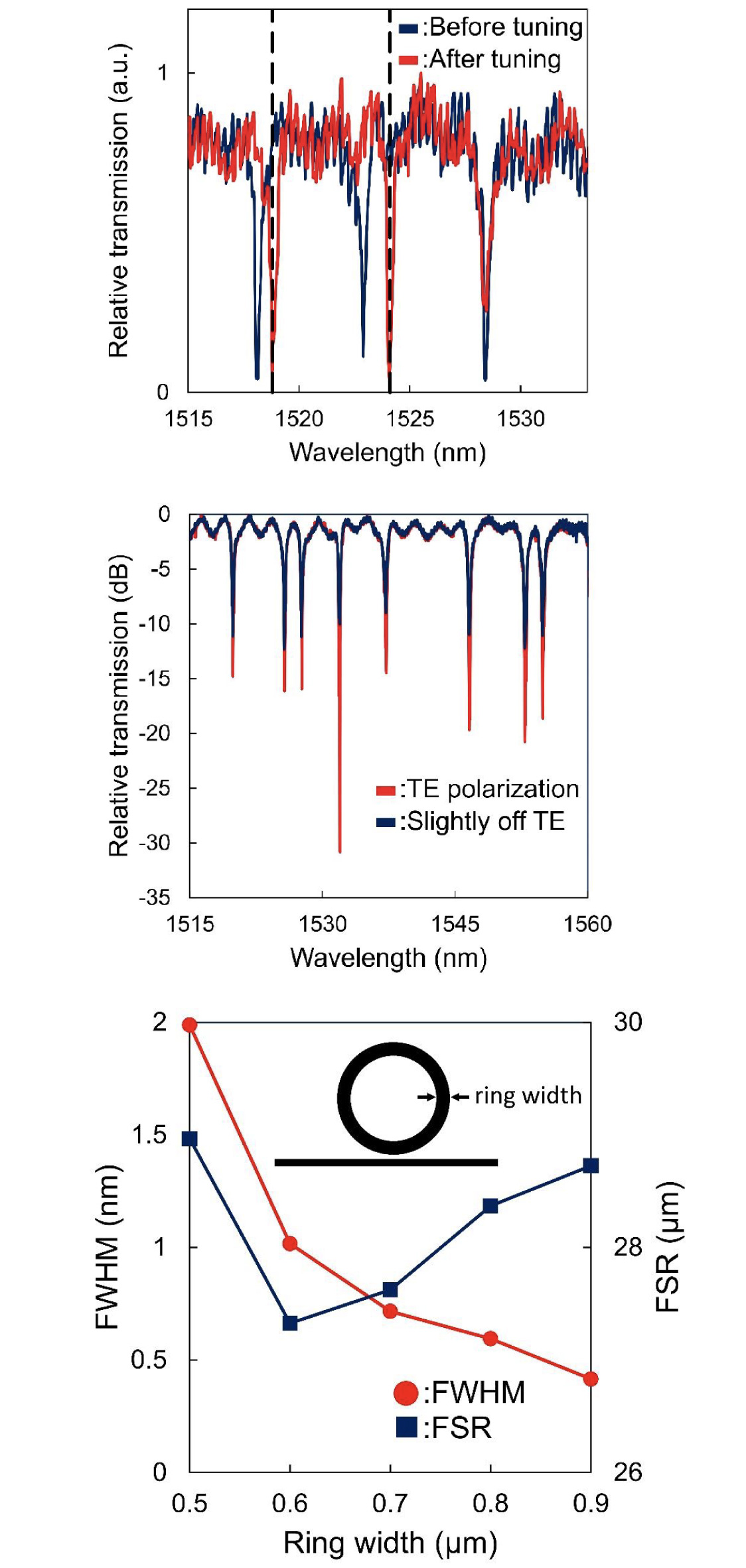
Figure 3: (Top) Laboratory-based measurements of suppression with ring resonator-based notch filters, before and after e-beam tuning. The first two notches are successfully tuned to OH emission lines at 1518.7 nm and 1524.1 nm (dashed lines), while the third reference notch is left untuned. (Middle) We also measured transmission of a five-ring device with input polarization set to transverse electric (TE), and then slightly off TE. On the TE polarization, multiple notches with 15 to 20dB of suppression can be seen, while on a state slightly off the TE polarization, the notches become shallower, but no transverse magnetic (TM) modes emerge. (Bottom) FWHM and FSR as a function of ring resonator width for notches near 1550nm. Inset shows schematic diagram of a ring resonator; increasing width greatly decreases the FWHM but only marginally affects the FSR.
1. Laboratory-based swept-wavelength measurements of rings coupled to waveguides on each device, allowing us to observe suppression at specific wavelengths compared to the nominal throughput at unsuppressed wavelengths.
2. Selection of devices with rings possessing the right characteristics for OH suppression, which are then integrated with existing astronomical instrumentation for on-sky engineering tests. Instead of a laser or swept-wave source for input, we point one end of a fiber directly at the sky, and then couple it to our photonic device as usual. On the output side, instead of coupling to a power-meter we couple to one of the infrared spectrographs available to us – for example, Lowell Observatory’s CSHELL [8], a variable resolution (R~4000-30000) spectrograph capable of receiving a fiber input.
By following these steps, we have been able to design, fabricate, and test devices with FSR>30 nm and with notch width<0.4nm. Additionally, we have tested methods in the laboratory that provide polarization-independent transmission. We have also performed swept-wave measurements of numerous notch filters both before and after e-beam tuning, with different input polarization settings, and with different physical characteristics of our rings (Figure 3)
Proposed Research: Engineering Methods for Advancing Ring Resonator Capabilities
Our previous research has given us a strong foundation on which to build, and the focus of our proposed research is to affirm (or potentially modify) the criteria from our earlier tests. Then, by fulfilling these updated criteria with our improved devices, we will prove the applicability of ring resonators for atmospheric OH suppression, thereby opening up new possibilities for infrared astronomical instrumentation.
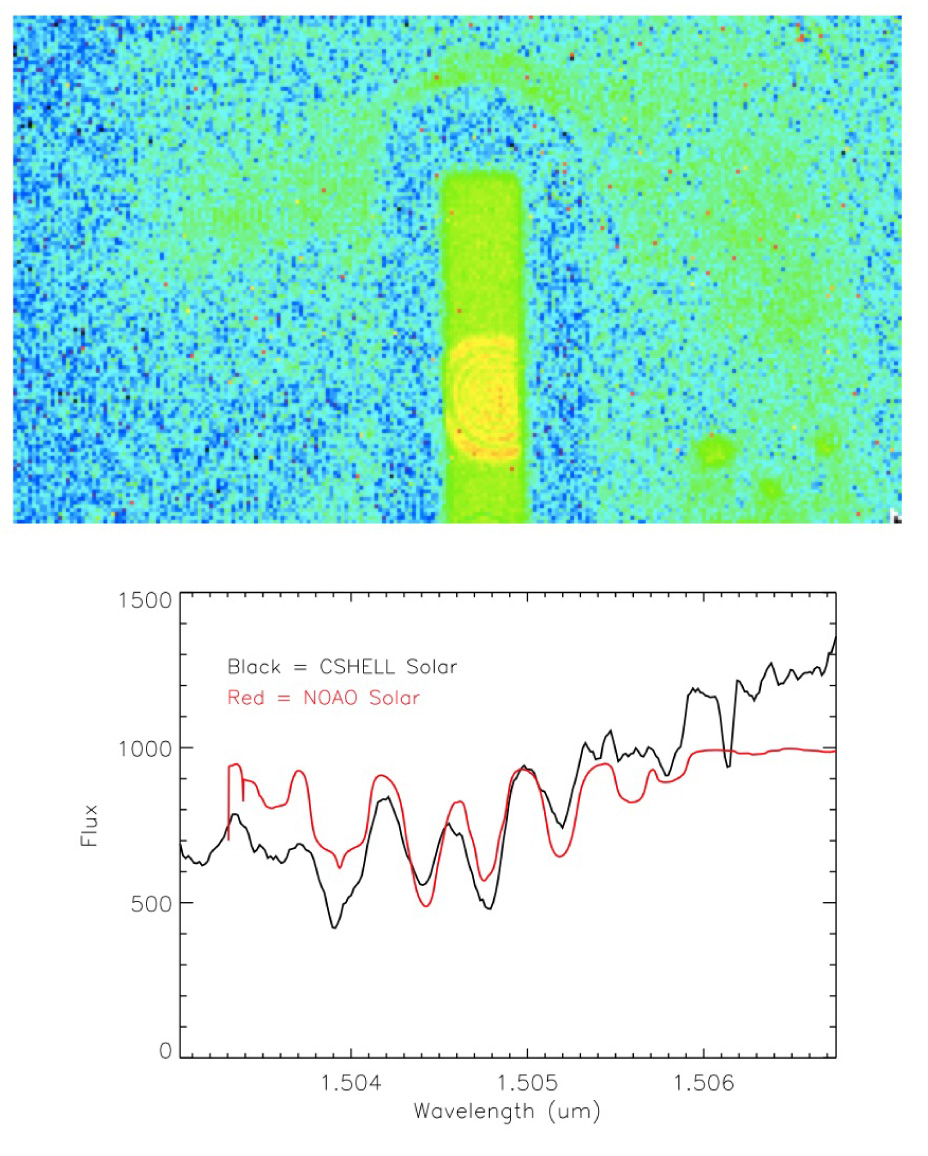
Figure 4: (Left) An image of the CSHELL slit (green vertical feature) with the input fiber (yellow circular feature) showing light collected from the sky and transmitted through our system. (Right) A narrow-band spectrum taken by CSHELL with its input fiber pointed directly at the daytime sky, showing the natural solar spectral features (black line) as compared to an NOAO references spectrum (red line).
The final step of our process advances our laboratory-based engineering results to the point where we are entering the realm of astronomical instrumentation. By feeding light from the sky through our ring-resonator-equipped (and thermally tuned) devices into the CSHELL spectrograph (Figure 4), we will directly measure the suppression of the OH lines, quantifying the increase in signal to noise that we theoretically predicted to be a factor of 5 or more.
Once this method proves successful, our ultimate goal is to design a prototype instrument that can be inserted into any fiber-based infrared instrument, making these advanced capabilities available to the entire astronomical community.
Strategic Applications to Astronomical Research
Photonic technologies hold significant potential for a wide variety of industrial and academic enterprises. Some fields have already incorporated such technologies into their regular operations. While some advances in instrumentation based upon photonics have been made in astronomy, there is much more that can be accomplished. As Pathways to Discovery in Astronomy and Astrophysics for the 2020s, the National Academies’ Decadal Survey for astronomy, states [5]:
Well established technologies developed in the past decades for fiber-optics telecommunications and other commercial applications are now emerging as potentially revolutionary strategies for a new generation of astronomical instruments.
It further recognizes that
Strengthening the coordination between the most active astrophotonics research groups in the United States would optimize resources and facilitate the passage from laboratory research to industrial partnership… Cost-savings for translational programs in astronomical applications can derive from the availability of past fundamental research, industrial prototypes, and available technological infrastructure for hardware production.
We will contribute to the realization of these goals with our proposed research by coordinating the efforts of multiple institutions, building upon our own past work, and leveraging available industrial infrastructure such as AIM Photonics.
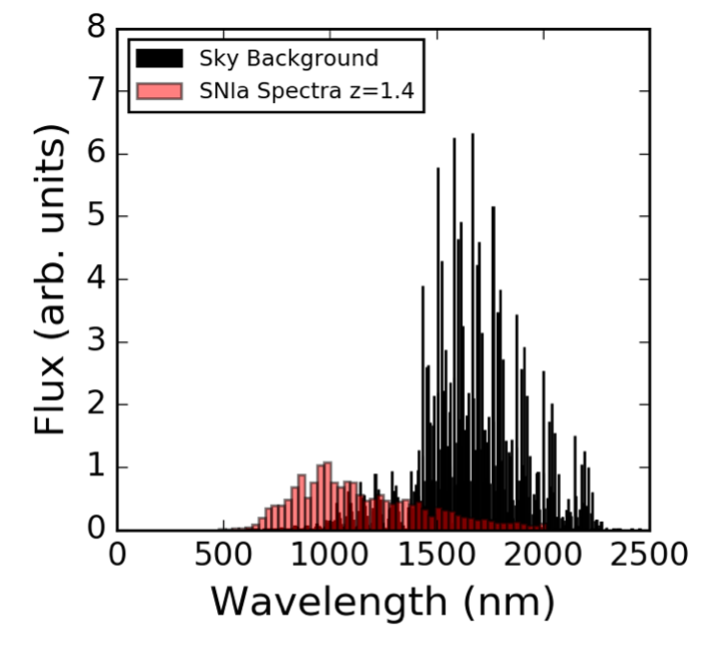
Figure 5: Nearby supernovae observable in visible wavelengths would be shifted toward the infrared (red curve) if they were more distant (redshift z=1.4). Observations of such supernovae are, at present, subject to overwhelming contamination from the OH emission spectrum (black lines). With OH suppression of just the most prominent lines in the H-band, the signal-to-noise of the astrophysical observations can be increased by up to a factor of five [7].
Apart from advancing the engineering and astronomical instrumentation capabilities of the photonic technologies now within our reach, our own scientific interests lie in applying ring-resonator-based OH suppression systems to observe the grandest size scales of the universe. Previously, supernova cosmology has focused on the “nearby” (redshift z< 1) universe, since spectral lines of supernovae at greater distances are redshifted into the infrared, where they run afoul of the atmospheric OH emission (Figure 5). However, with OH suppression, supernovae could be effectively and efficiently measured to much greater distances, thereby giving astronomers a longer lever-arm in constraining cosmological parameters, and perhaps even giving them an edge in elucidating the nature of dark matter and dark energy.